Showcase Research
The Mars Sample Return Campaign is an international collaboration involving NASA, ESA and reasearchers from around the world.
Meet the Martians
Click on an image to find out more about some of the people who study Mars and are working to make Mars Sample Return a reality.



Analysis of the Returned Samples on Earth
Analysis of the samples brought back from Mars will be an international effort and will include scientists from a range of disciplines. Studies of the returned samples will provide insights into their texture, mineralogy, structure and chemistry, as well as the presence of any biosignatures.

Analysis techniques for meteorites and other extraterrestrial material retrieved by missions typically fall into three categories: imaging, non-destructive, and destructive.
Non-destructive techniques preserve the sample, whereas destructive techniques require that some of the sample is destroyed in the process of analysis. Scientists always try to use non-destructive methods, where possible, to preserve samples. However, some destructive techniques can give unique and powerful insights into the chemistry of the samples. Destructive analysis techniques are optimised to require the smallest possible sample mass, so that only a small piece of the whole sample needs to be lost.
A portion of each of the samples will be kept untouched so they can be analysed at a later date when there have been advancements in technologies and new scientific questions come up. Scientists are still making new discoveries today on lunar samples brought back by the Apollo astronauts more than 50 years ago, so samples returned from Mars are likely to produce groundbreaking science for decades to come.
Deep Dive into Analysis Techniques
Imaging techniques
First, the returned sample tubes containing martian material are likely to be scanned using X-ray micro-computed tomography (micro-CT). This provides textural and compositional information on samples and can be conducted when the samples are still sealed in their containers and pristine. Once the samples have been prepared and distributed for analyses, imaging with instruments such as optical microscopes and Scanning Electron Microscopes will provide detailed textural context and information on the mineralogy of the samples.
Non-Destructive Techniques
A range of non-destructive analysis techniques offer different perspectives on the samples.
- Scanning Electron Microscopy can provide high-resolution images of samples and the surface topography of samples, as well as provide qualitative chemical compositions
- Electron Microprobe analysis is a quantitative method of determining the chemistry of samples
- Raman Spectroscopy uses a laser to vibrate molecules within samples to provide information on the mineralogy and detect organic molecules.
Destructive Techniques
Mass spectrometry measures the mass of molecules in a sample by turning them into ions (charged particles). Mass spectrometry is used in the radiometric dating of rocks, which will be an important part of the analyses of the returned samples. Mass spectrometry can also be used to gain more insight into the chemical composition of the samples, such as isotopic concentrations and contents of rare earth elements. Types of mass spectrometry include:
- Secondary Ion Mass Spectrometry
- Nano-Secondary Ion Mass Spectrometry
- Inductively Coupled Plasma Mass Spectrometry
- Noble Gas Mass Spectrometry.
The actual preparation of samples for some techniques may be destructive. X-Ray Diffraction techniques require the samples to be in a fine powder form and Transmission Electron Microscopy requires samples to be extremely thin (between 10 and 100 nanometres thick).
Other destructive techniques are needed to look for key molecules required for life and to determine if biosignatures are present. These techniques include:
- Gas Chromatography Mass Spectrometry
- Liquid Chromatography Mass Spectrometry
- Infrared Spectroscopy
- Ultraviolet Spectroscopy
- X-Ray Absorption Near Edge Spectroscopy.
Importance of Returned Samples
The returned samples will enable major advances in our understanding of the processes, chemistry, timing, and biological potential of Jezero Crater and other sedimentary systems on Mars. They will also help address questions about the atmosphere, global evolution and geology of Mars.
Deep Dive into the Importance of Returned Samples
Increasing the Diversity of Mars Rocks for Analysis on Earth
We know from previous and current Mars missions that there is more diversity in rocks on Mars than is represented in worldwide collections of martian meteorites. Fragile sedimentary rocks may not be able to survive being ejected from the martian surface into space, so we are unlikely to find martian meteorites of this type on Earth. The only way to examine sedimentary rocks from the martian surface in more detail is to collect them and return them to Earth for analysis.
Bringing a diverse sample suite of rocks (igneous, sedimentary, and regolith samples) back to Earth will allow scientists to better understand the geology and the evolution of Mars.
Dating the Exact Ages of Martian Rocks
Scientists count the number, size, density and morphology of craters across the martian surface to create a timeline of which surfaces formed first and which formed later. This method is based on the assumption that any new martian surfaces will have no impact craters but, as time goes on, craters will accumulate.
NASA’s Curiosity rover carries a spectrometer to date rocks on Mars by measuring isotopes. In 2013, the Sheepbed mudstone in Gale Crater became the first rock on Mars to be dated. Curiosity determined that from the potassium and argon isotope content that the rock was in the range of 3.86 to 4.56 billion years old.
The ages of martian meteorites can be more precisely dated in laboratories on Earth. However, as scientists do not know their exact places of origin on Mars, their age cannot be accurately linked to a surface location. Once on Earth, the igneous rocks collected by Perseverance will be dated by scientists in specialised laboratories. Since the sample locations of these rocks are known, precise ages may be given to those areas of the martian surfaces. This will provide a calibration for the crater counting method on Mars.
Detecting Potential Past Life
Perseverance is collecting rocks that are likely to contain signs of whether ancient life and past habitable environments ever existed on Mars. Sedimentary rocks are the best candidates to help achieve this goal, as they can contain sulphates, carbonates, organics and clays that are good at preserving biosignatures.
Investigating Mars’s Wet Past
Some of the samples collected by Perseverance contain direct evidence of chemical changes that occurred in the presence of water. Analysing these samples will help scientists understand the timing and nature of water that once flowed on the surface of Mars.
Sniffing the Martian Atmosphere
As well as rock and soil samples, Perseverance is collecting samples of gases in the martian atmosphere.
It is likely that all the sample tubes that contain rock cores and regolith will also contain some martian atmosphere. However, dedicated atmospheric samples will not have interacted with the rock material in the sample tube and will, therefore, be a truer representation of the current martian atmosphere. Scientists will analyse the samples to determine an accurate composition of the martian atmosphere. Measurements of noble gases and isotopes will provide information on the formation, outgassing and evolution of the atmosphere of Mars.
Supporting Future Missions
The returned samples will help scientists design future missions and equipment that can operate on martian surface efficiently and safely to support human explorers.
Martian dust is thought to be a potential hazard, as it could cause abrasion and degrade exposed surfaces, such as spacesuits and systems that contain joints and seals. Although no martian dust will be specifically collected and returned to Earth, cached sample tubes will be covered in dust from the martian surface. If these back-up samples are returned, the exterior of the tubes could provide insights into the properties of airfall dust and the potential impacts on spacesuits and engineering systems.
Importantly, the returned samples will help to determine if Mars offers any potential biological hazards and threats to the health of human explorers. If so, it will have a significant effect on plans for the human exploration of Mars.
Martian Analogues

Earth hosts a range of ‘analogue’ environments that mimic conditions that exist elsewhere in our Solar System, including Mars. Martian analogues may be linked to be a specific geological process, the way minerals are transformed, or environmental conditions.
Visiting martian analogues is an important way in which scientists can advance our understanding of Mars. During field trips, researchers can use all their senses and state-of-the-art equipment to explore and characterise the environments in-situ and collect samples for further analysis in the laboratory. Using martian analogues can help fill in gaps in our understanding of the martian environment, its evolution, and potential habitability.
Deep Dive: Mars Analogues
Jezero Crater Analogues
The Mars Sample Return Rock Sample Team organised a field trip in October 2023 to collect analogue rocks from five sites on Earth:
- Salton Sea, California, USA (a terrestrial analogue for the Jezero Delta sedimentary rocks)
- Ridge Basin, California, USA (a terrestrial analogue for the Jezero Delta sedimentary rocks)
- Lambahraun, Iceland (a terrestrial analogue for martian regolith and granular material)
- Isle of Rum, Scotland (a terrestrial analogue for the Séítah Formation in Jezero Crater)
- Hart Mountain, Oregon, USA (a terrestrial analogue sample for the Máaz Formation in Jezero Crater).
The Haughton Mars Project
The Haughton Mars Project, led by NASA’s Ames Research Centre, organises field trips to the Haughton meteorite crater on the uninhabited Devon Island in Canada.
- Devon Island’s cold climate, terrain and remoteness make it a good analogue for Mars.
- The Haughton Mars Project develops and tests new technologies that could be used in future robotic and human missions to Mars.
Europlanet Mars Analogues
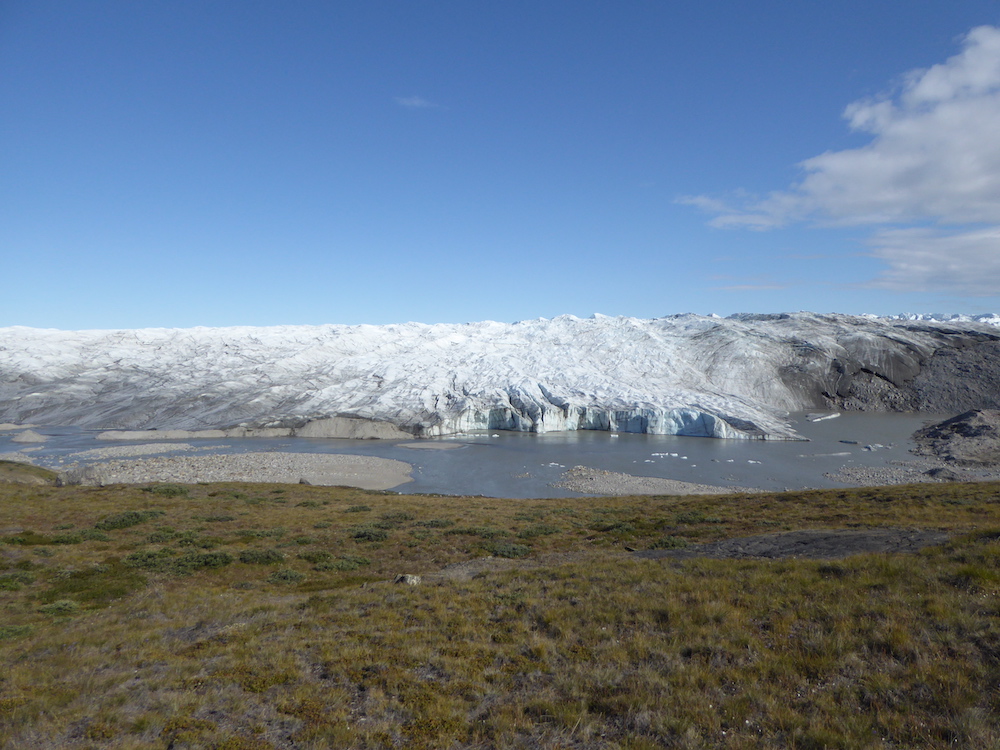

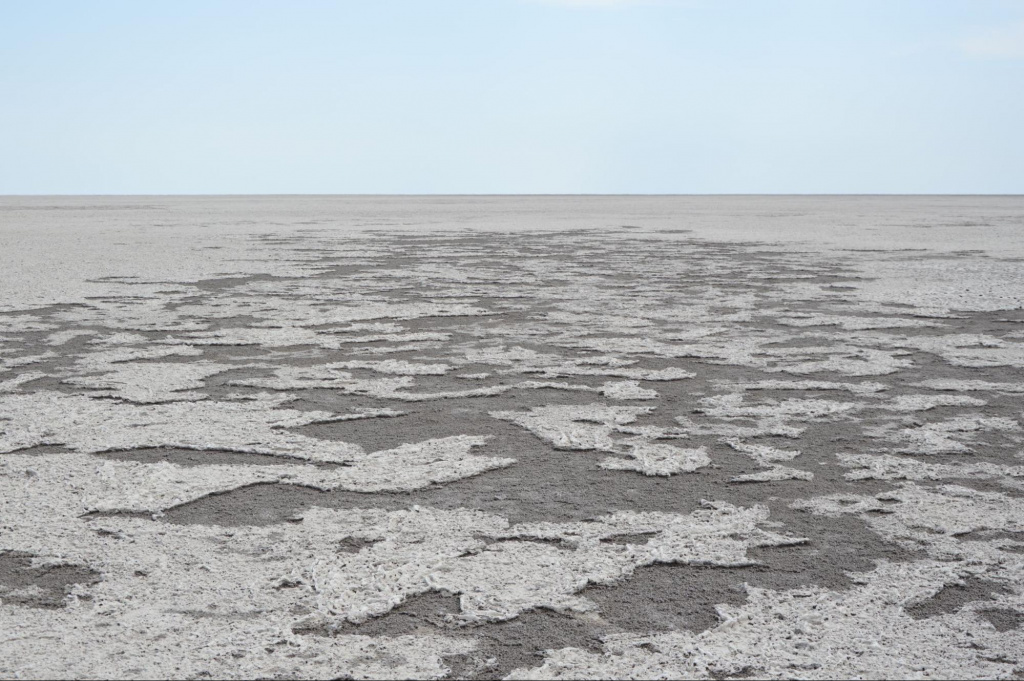

International researchers are supported to visit Mars analogue sites through the Europlanet project, including:
- The volcanic terrains of Iceland (terrestrial analogues of the igneous rocks collected by Perseverance).
- The acidic river of Rio Tinto (where bacteria have adapted to the unusual, extreme chemical conditions).
- The Makgadikgadi salt pans of Botswana (where bacteria have adapted to the extreme saline conditions).
- Cold, dry lakes and glaciers of the Argentina Puna region, the Qaidam Basin desert in the north of the Tibetan Plateau, and the glacial icesheet of Kangerlussuaq, Greenland (analogues for Mars polar regions).
Europlanet also supports visits to laboratory facilities that can recreate atmospheres, pressures and temperatures for scientists to run experiments under martian conditions. These include:
- The Mars Chamber at the Open University in Milton Keynes, UK, which can recreate conditions on the surface of Mars for experiments and to test equipment.
- The Planetary Environment Facility at Aarhus University in Denmark is cryogenic (cold) wind tunnel that can be used to study how sand, ice and dust move around under martian conditions.
The Planetary Terrestrial Analogues Library
The Planetary Terrestrial Analogues Library is a collection of Earth rocks that have compositions comparable to the rocks on Mars.
- The collection currently contains 102 igneous and sedimentary rocks as well as regolith collected from around the world.
- Scientists use the library to calibrate and interpret data from spectroscopy instruments that are onboard Mars orbiters to improve mineralogical analysis of rocks present at the martian surface.
- The collection supports studies of alteration and erosion of rocks on the surface of Mars.
References
References:
Williams, N. R., Golombek, M. P., Do, S., Claef, F., Lethcoe, H., Cameron, M., Trussell, A., Brooks, C., Russo, F., Deahn, M., Morris, M., Hibbard, S., Heverly, M., Spencer, D., Fosse, E. and Maki, J. (2023). Mars Sample Return “Three Forks” Landing and Depot Site Selections. 54th Lunar and Planetary Science Conference, Abstract # 2618.
Maki, J. N., Farley, K., Stack, K., Calef, F., Williams, N., Bell, J. F., Herd, C. D. K., Wadhwa, M. and Brown, A. (2023). The Mars 2020 Three Forks Sample Depot. 54th Lunar and Planetary Science Conference, Abstract # 2875.
https://mars.nasa.gov/resources/27268/perseverances-three-forks-sample-depot-map/
https://www.nasa.gov/feature/jpl/nasa-s-perseverance-rover-shows-off-collection-of-mars-samples
https://www.jpl.nasa.gov/news/nasas-perseverance-rover-shows-off-collection-of-mars-samples
NASA’s Perseverance Rover Deposits First Sample on Mars Surface – NASA Mars Exploration
Mars Rock Samples – NASA Mars Exploration
References: Return to Earth
Sample Retrieval Lander – NASA Mars
Sample Recovery Helicopters – NASA Mars
Mars Ascent Vehicle – NASA Mars
Earth Return Orbiter – ESA – NASA Mars
https://www.jpl.nasa.gov/missions/mars-sample-return-msr
https://mars.nasa.gov/msr/#Sample-Retrieval
https://mars.nasa.gov/resources/26895/mars-sample-return-concept-illustration/
https://mars.nasa.gov/internal_resources/1305/