Educational Resources – The Mars Collection
Europlanet has produced a set of school resources exploring the possibilities of life on the Red Planet.
The resources have been produced to be easily translatable in order to facilitate ease of dissemination across Europe and beyond. The resources link areas of the curriculum with research into past and present conditions on Mars and how we can study these conditions via planetary field analogue sites here on Earth. The project covers a range of topics, from geoscience and volcanoes, to pH and even mineral deposition, all viewed through an astrobiological lens.
Italian versions of the resources with video lessons produced by EduINAF are now available at: Terra chiama Marte.
English versions are accompanied by video and webinar training sessions to support teachers wishing to engage with these resources.
Resources
Presentations
1. Extremophiles
- English PPT | PDF
- French PPT | PDF
- Greek PPT | PDF
- Portuguese PPT | PDF
- Russian PPT | PDF
- Spanish PPT | PDF
4. Martian Chemistry
7. pH of Mars
2. A Brief History of Mars
5. Brines on Mars
Teachers’ Notes
1. Extremophiles
- English Word | PDF
- French Word | PDF
- Greek Word | PDF
- Portuguese Word | PDF
- Russian Word | PDF
- Spanish Word | PDF
4. Martian Chemistry
7. pH of Mars
2. A Brief History of Mars
5. Brines on Mars
Europlanet 2024 RI Planetary Field Analogue Sites
The resources are linked to six sites on Earth that resemble other planets (planetary field analogues) that Europlanet 2024 RI enables scientists and engineers to visit to carry out research projects.
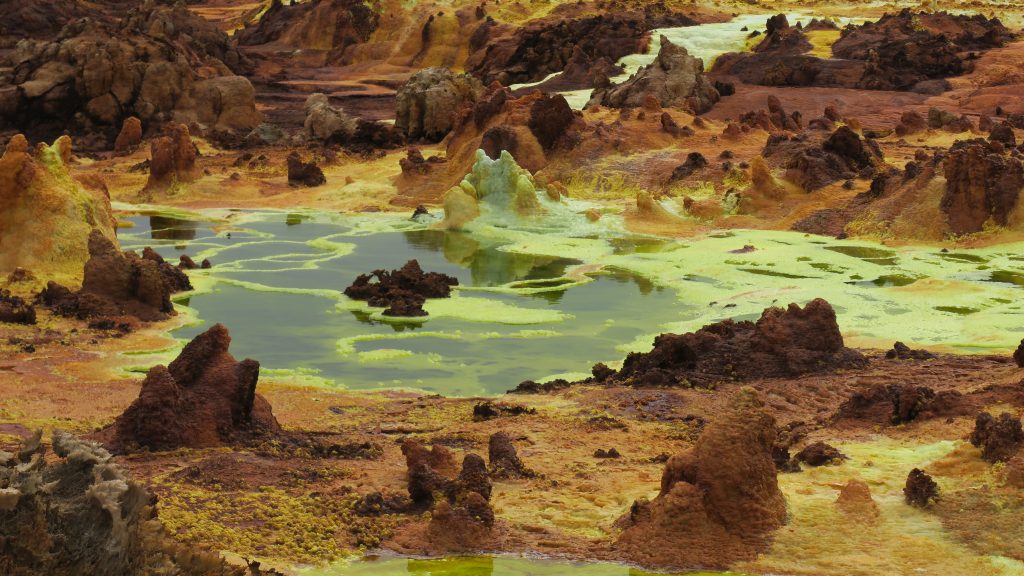

Danakil Depression
(See Resource 2. Brief History of Mars)
Tharsis is a vast volcanic plateau centred near the equator in the western hemisphere of Mars. The region is home to three enormous shield volcanoes: Arsia Mons, Pavonis Mons, and Ascraeus Mons, which are collectively known as the Tharsis Montes, as well the largest known volcano in the Solar System, Olympus Mons.
An effective analogue for the Tharsis region of Mars is the Danakil Depression in Ethiopia. The Danakil Depression lies at the triple junction of three tectonic plates and has a complex geological history. It has developed as a result of Africa and Asia moving apart, causing rifting and volcanic activity. Erosion, inundation by the sea and the rising and falling of the ground have all played their part in the formation of this depression. Sedimentary rocks, such as sandstone and limestone, are overlain by basalt that has resulted from extensive lava flows.
Iceland Field Sites
(See Resource 3. Volcanoes on Mars)
The largest volcano in the Solar System can be found on Mars. This is called Olympus Mons and is a shield volcano. It is 25km tall, with a diameter of 624km. Olympus Mons is wide enough to stretch from the West Coast of France to its Eastern border.
Olympus Mons is thought to have achieved its size due to the lack of tectonic movement on Mars, allowing multiple eruptions from a single fissure. With each eruption and subsequent solidification of the lava flows, the volcano increased in size. These multiple eruptions have left a distinctive stripped pattern upon the volcano, with each stripe showing a separate eruption.
Of course, we can’t study Olympus Mons close up, but here on Earth we use planetary field analogue sites, such as the volcanically active environment of Iceland.
Iceland owes much of its landscape to its volcanic activities, making it a fascinating case study in volcanic processes. Iceland has numerous geothermal fields. Some of these are close to Reykjavik, such as Krýsuvík. All of these fields have different geothermal wells: pools of water warmed up by the Earth’s underground heat. The heating causes all wells to have temperatures ranging from 60 to 95°C.
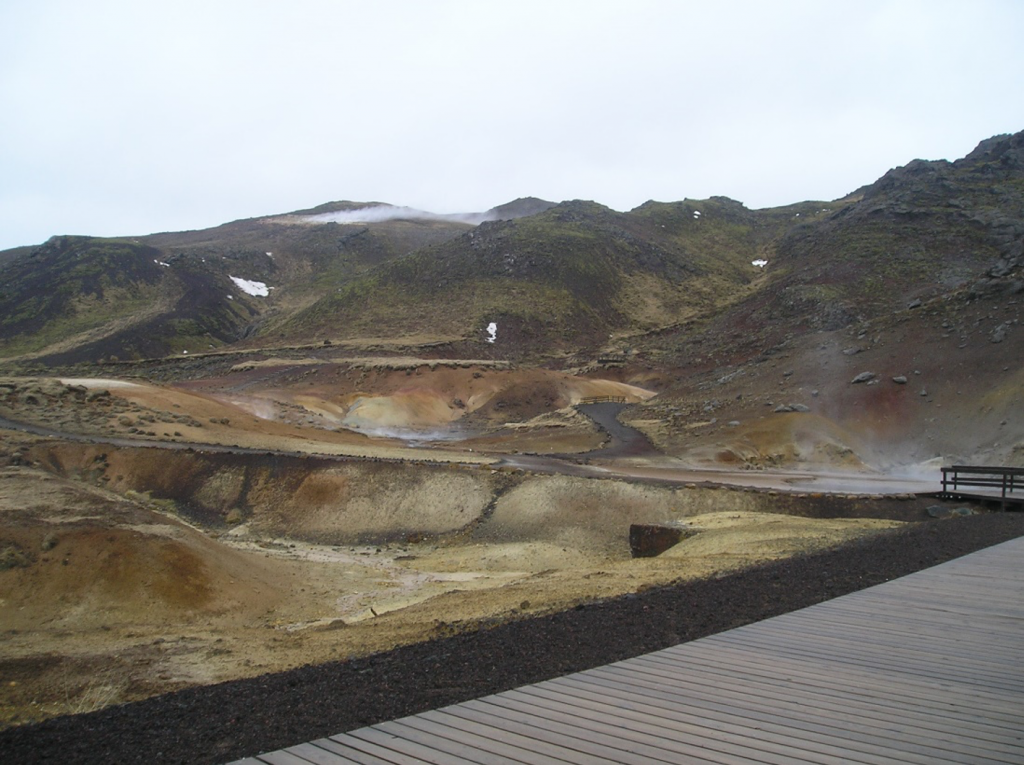

Kangerlussuaq, Greenland
(See Resource 4. Martian Chemistry)
Some researchers think that there may be lakes of water beneath the icy poles of Mars
One way that researchers are hoping to gain more information on this possibility is by exploring analogues here on Earth. One of the best analogues for the martian poles are areas such as Kangerlussuaq in Greenland. Greenland is the world’s largest island and over three-quarters of its surface is covered by the only permanent ice sheet outside of Antarctica. It is one of the few truly extreme cryogenic environments on Earth and yet is relatively accessible.
Kangerlussuaq, on the west coast of Greenland, is one of the most accessible regions of the island (with an international airport). The Kangerlussuaq planetary analogue site gives access to the glacial ice sheet and vast regions of permafrost.

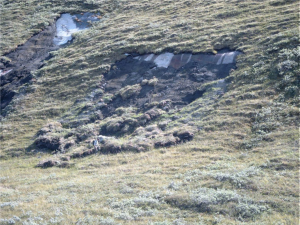


Argentinian Andes
(See Resource 5. Brines on Mars)
Researchers believe that there would have been saturated brine lakes on the surface of Mars at one point during the planet’s history. To investigate whether these lakes would have been viable for early Martian life, analogue sites here on Earth can be studied.
One such analogue site is Laguna Negra, a shallow lake that lies on the Puna Plateau in Northern Argentina. This environment is saturated with calcium chloride salts and presents a very harsh environment for life.
The presence of microbial life has been detected within the Laguna Negra; these life forms are halophilic, thriving in salt rich environments.
Makgadikgadi Salt Pans
(See Resource 6. Evaporation and States of Matter)
The Makgadikgadi Salt Pans in Botswana are a huge expanse of salt that has become very valuable in the study of microbiology in areas of high salinity.
Desiccation (a state of extreme dryness) is a common stress that bacteria face in the natural environment. Therefore, they have developed a variety of protective mechanisms to mitigate the damage caused by water loss. Some species have developed mechanisms that either help protect susceptible cellular components from damage, or that sequester water in an attempt to avoid dehydration. This has led some researchers to believe that life could survive the extreme environments such as high salinity found on Mars.


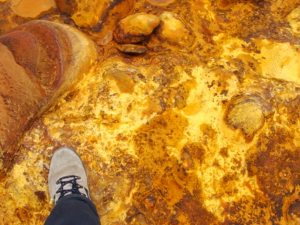
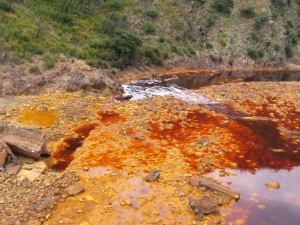
Rio Tinto
(See Resource 7. pH of Mars)
There are areas on Earth with extreme pH readings. One such location is the Rio Tinto river in Spain. The pH of the Rio Tinto ranges between 2.4 and 4.0 meaning that it is well in the range of being a strong acid. This is due to rock-water-biology interactions. Ferrous sulphate has a pH of approximately 2 and, as it oxidises, it precipitates iron. The iron gives the Rio Tinto its distinctive red colouration.
Although this was detrimental to multicellular organisms within the environment, it gives a very interesting opportunity for microbiology. Both eukaryotic and prokaryotic microorganisms have been observed in this extreme environment. The Rio Tinto planetary field analogue site can help inform us on the prospects of life in extreme environments elsewhere in the Solar System.
Interviews@EPSC – Mars Exploration
Ever wondered what it’s like to be involved in a mission to Mars? At the Europlanet Science Congress in September 2020, we interviewed José Antonio Rodriguez Manfredi (Centro de Astrobiologia in Madrid) about his role as Principal Investigator of the MEDA environmental sensor on NASA’s Perseverance Rover, and Elliot Sefton-Nash (European Space Agency) about the ExoMars Rosalind Franklin Rover.
Back to Educational Resources